Advanced pharmaceutical bulletin. 13(1):36-47.
doi: 10.34172/apb.2023.004
Review Article
Toll-Like Receptor 4 (TLR4) and AMPK Relevance in Cardiovascular Disease
Haleh Vaez 1, *
, Hamid Soraya 2
, Alireza Garjani 1, Tooba Gholikhani 3, 4
Author information:
1Department of Pharmacology and Toxicology, Faculty of Pharmacy, Tabriz University of Medical Sciences, Tabriz, Iran.
2Department of Pharmacology, Faculty of Pharmacy, Urmia University of Medical Sciences, Urmia, Iran.
3Student Research Committee, Faculty of Pharmacy, Tabriz University of Medical Sciences, Tabriz, Iran.
4Nanora Pharmaceuticals Ltd, Tabriz, Iran.
*
Corresponding Author: Haleh Vaez, Tel:+984133344798, Fax:+984133344798, Email:
vaezh@tbzmed.ac.ir
Abstract
Toll-like receptors (TLRs) are essential receptors of the innate immune system, playing a significant role in cardiovascular diseases. TLR4, with the highest expression among TLRs in the heart, has been investigated extensively for its critical role in different myocardial inflammatory conditions. Studies suggest that inhibition of TLR4 signaling pathways reduces inflammatory responses and even prevents additional injuries to the already damaged myocardium. Recent research results have led to a hypothesis that there may be a relation between TLR4 expression and 5' adenosine monophosphate-activated protein kinase (AMPK) signaling in various inflammatory conditions, including cardiovascular diseases. AMPK, as a cellular energy sensor, has been reported to show anti-inflammatory effects in various models of inflammatory diseases. AMPK, in addition to its physiological acts in the heart, plays an essential role in myocardial ischemia and hypoxia by activating various energy production pathways. Herein we will discuss the role of TLR4 and AMPK in cardiovascular diseases and a possible relation between TLRs and AMPK as a novel therapeutic target. In our opinion, AMPK-related TLR modulators will find application in treating different immune-mediated inflammatory disorders, especially inflammatory cardiac diseases, and present an option that will be widely used in clinical practice in the future.
Keywords: AMPK, Cardiovascular disease, Inflammation, TLRs
Copyright and License Information
©2023 The Authors.
This is an Open Access article distributed under the terms of the Creative Commons Attribution (CC BY), which permits unrestricted use, distribution, and reproduction in any medium, as long as the original authors and source are cited. No permission is required from the authors or the publishers.
Introduction
Inflammation has long been associated with many diseases, including diabetes, cancer, arthritis, and cardiovascular complications. It is triggered when the immune system, including bone marrow-derived cells like monocytes and macrophages and non-bone marrow-derived cells, detects infection or tissue injury as the body’s first-line defense. Since TLRs are expressed in almost all heart cells, their involvement in developing cardiovascular diseases (CVDs) is evident. However, recent regard to the importance of innate immunity leads to new investigation areas. It may result in identifying potential novel therapeutic targets for inflammatory diseases treatment.
Toll-like receptors (TLRs) are essential receptors of the innate immune system, prerequisites for inducing adaptive immune responses against pathogens. They have been termed pattern recognition receptors that detect conserved motifs on pathogens, pathogen-associated molecular patterns (PAMPs), lipopolysaccharide (LPS) of gram-negative bacteria, or viral double-stranded RNAs.1 Furthermore, TLRs can also recognize damage-associated molecular patterns or danger-associated molecular patterns (DAMPs), which are endogenous host material propagated during cellular stress or death.2 Thereby, TLRs play a role in different steps of recognizing through innate immunity and regulate the generation of adaptive immunity by forming immunological memory and polarization of antigen responses and responses to tissue damage.3
Although inflammation is a crucial survival mechanism, it can induce severe damage to the tissue in case of being inordinate or turned into a chronic state. Hence, the identification and studying the immune regulators is of value. The 5’ adenosine monophosphate-activated protein kinase (AMPK) is a novel immune modulator with therapeutic efficacy in different inflammatory conditions.4,5 There are several publications describing the role of AMPK activation in inhibiting inflammatory responses induced by different stimulating factors like acute and chronic colitis,6 cystic fibrosis,7 autoimmune encephalomyelitis,8 and pro-inflammatory effects after lung injury.9 The results of several studies suggest a hypothesis that there may be a relation between AMPK and TLR4 signaling in various inflammatory conditions.10-14 This review will summarize the role of TLR4 in CVDs and elaborate on the hypothesis that the AMPK involve in improving the immune responses following tissue injury and the inflammatory process.
TLRs
The Toll protein was first discovered as the receptor responsible for early embryonic development in Drosophila fruit fly.15,16 Medzhitov et al17 explained that the human homolog of Drosophila Toll protein could trigger an innate immune response. To this day, 11 human and 13 mice TLRs have been identified. TLR-1, TLR-2, TLR-4, TLR-5, TLR-6, TLR-10, TLR-11, and TLR-12 are expressed on the cell surface and recognize components derived from microbes such as lipids, lipoproteins, and proteins. Whereas TLR-3, TLR-7, TLR-8, TLR-9, and probably TLR-13 of mice are intracellular proteins that detect nucleic acids. TLRs 11 and 13 are only expressed in mice.18
TLRs are expressed in various types of tissues, including the heart, brain, kidney, liver, lung, small intestine, spinal cord, spleen, and reproductive organs.19 Since among all TLRs, TLR4 is the most expressed protein in the heart, as a potential and significant receptor has been extensively investigated for its critical role in different myocardial inflammatory conditions, including endotoxemia,20,21 myocarditis,22 myocardial infarction (MI),23 ischemia/reperfusion (I/R) injuries,24 heart failure,25 aortic valve diseases,26 atherosclerosis,27 and hypertension.28
TLR signaling
TLR4 is well known as the receptor for LPS, a gram-negative bacteria membrane component of the outer membrane.29 Fusing of TLR4 with myeloid differentiation 2 (MD2) on the cell surface is essential for ligand-induced signaling activation.30 Several proteins modulate TLR signaling pathways that improve LPS recognition: LPS-binding protein (LBP) and CD14, a glycophosphatidylinositol-anchored protein.31,32
Upon activation and ligand recognition, TLR4 induce the assembly of homodimerization. Depending on the adaptor usage, the intracellular signaling pathways are divided into two pathways as shown in Figure 1: the myeloid differentiation factor 88 (MyD88)- dependent and the MyD88-independent pathways.33
Figure 1.
Two distinct signaling pathways after TLR4 activation. MyD88-dependent and the MyD88-independent pathways. (A) MyD88 engages IL-1 receptor-associated kinases (IRAKs), followed by interplay with TNF-receptor-associated factor 6 (TRAF6), and the downriver stimulation of transforming growth factor 𝛽-activated kinase 1 (TAK1). Then, activated TAK1 phosphorylates IkB kinase complex (IKKβ), directing the phosphorylation and degradation of I-κB, which discharge nuclear factor-κB (NF-𝜅B) and results in the nuclear translocation and DNA attaching of NF-κB where it stimulates multiple gene expression. (B) MyD88-independent pathway involves the recruitment of adaptor proteins TRIF and TRAM, and the evocation of IFN regulatory factor 3 (IRF3) nuclear translocation, facilitated by TBK1 (tank-binding kinase 1) and IKK𝜀 ( inhibitory κB (IκB) kinase ε) which finally result in production of type I IFNs and proinflammatory cytokines.
Figure 1.
Two distinct signaling pathways after TLR4 activation. MyD88-dependent and the MyD88-independent pathways. (A) MyD88 engages IL-1 receptor-associated kinases (IRAKs), followed by interplay with TNF-receptor-associated factor 6 (TRAF6), and the downriver stimulation of transforming growth factor 𝛽-activated kinase 1 (TAK1). Then, activated TAK1 phosphorylates IkB kinase complex (IKKβ), directing the phosphorylation and degradation of I-κB, which discharge nuclear factor-κB (NF-𝜅B) and results in the nuclear translocation and DNA attaching of NF-κB where it stimulates multiple gene expression. (B) MyD88-independent pathway involves the recruitment of adaptor proteins TRIF and TRAM, and the evocation of IFN regulatory factor 3 (IRF3) nuclear translocation, facilitated by TBK1 (tank-binding kinase 1) and IKK𝜀 ( inhibitory κB (IκB) kinase ε) which finally result in production of type I IFNs and proinflammatory cytokines.
MyD88 associates with all TLR types except TLR3. MyD88 engages IL-1 receptor-associated kinases (IRAKs), followed by interaction with TNF-receptor-associated factor 6 (TRAF6), and the downstream stimulation of transforming growth factor 𝛽-activated kinase 1 (TAK1), facilitated by the adaptor proteins, TAK1-binding protein 2 and TAK1- binding protein 3 (TAB2 and TAB3). Then, activated TAK1 phosphorylates IkB kinase complex (IKKβ), directing the phosphorylation and degradation of I-κB, which discharge nuclear factor-𝜅B (NF-𝜅B) and result in the nuclear translocation and DNA attaching of NF-κB where it stimulates multiple gene expressions.34
MyD88-independent pathways or Trif-dependent pathway (Toll/interleukin-1 receptor-like (TIR) domain-containing adaptor inducing IFN-β) is utilized by TLR3 and TLR4. It involves the recruitment of adaptor proteins TRIF and TRAM (TRIF-related adaptor molecule), activation of TNF receptor-associated factor 3 (TRAF3), and the evocation of IFN regulatory factor 3 (IRF3) nuclear translocation, facilitated by tank-binding kinase 1 (TBK) and IKK𝜀 which finally result in the production of type I IFNs.18,35
TLRs and inflammation
The production of inflammatory cytokines and chemokines directly affects TLRs stimulation upon ligand encountering, which directs various inflammatory cells into the ligand presented sites. The appropriate inflammatory response induced by TLRs is required to function both innate and adaptive immune responses. However, its inordinate signaling may result in autoimmune/inflammatory diseases.36 Thereby, TLR signaling needs to be strictly adjusted.
Cells have obtained a series of strategies for negative regulation of TLR signaling at multiple steps. Complex regulation at several levels varying from extracellular soluble TLRs to transmembrane and intracellular inhibitors such as those that retain the TIR domain indicates that regulation can be acquired by a cascade of regulators. This declares that a specific inhibitor may be integral but not sufficient to adjust the pathway.37,38
A negative regulator of TLR signaling is a transcriptional repressor, the orphan nuclear receptor small heterodimer partner (SHP), which abundant LPS-induced expression of its lead-in inhibition of TLR signaling in AMPK dependent pathway.39
TLR4 and CVDs
Besides immune cells, TLRs are expressed in endothelial cells, smooth muscle cells, and cardiomyocytes.40 As previously mentioned, TLR4 is the most studied TLRs with the highest expression level in the heart. Although cardioprotective effects of short-term activation of this receptor have been demonstrated in isolated perfused hearts,41 studies have reported its implication in myocarditis, MI, myocardial I/R injury, heart failure, cardiac arrhythmias, cardiac valve diseases, atherosclerosis, and hypertension.40,42 TLR4 is involved in the etiology of hypertension and introduced as a possible novel therapeutic target for hypertension treatment. Recent studies have been suggested a cross-talk between angiotensin II (Ang II) and TLR4 in hypertension.43 It was reported that Ang II upregulated the TLR4 on mesangial cells and finally contributed to renal inflammation and fibrosis.44,45 Zhang et al46 demonstrated that TLR4 antagonist, TAK-242, reversed aldosterone-induced cardiac and renal injury. An increased level of TLR4 expression in advanced heart failure has been reported previously, and inhibition of this receptor reduced the heart failure progression.40,47 Cardiomyocyte necrosis due to MI releases various endogenous DAMPs associated with profound TLR4 activation and inflammatory responses, leading to additional damage to the injured myocardium.48 Soraya et al13 reported cardioprotective effects through suppression of TLR4 expression in MI.
AMPK
AMPK, a serine/threonine kinase, is a highly conserved sensor of cellular energy balance and is activated under the condition of ATP depletion induced by any cellular stresses such as hypoxia, glucose deprivation, or metabolic prevention of ATP biogenesis, exercise, osmotic stress, and oxidative stress.49 AMPK consists of catalytic α units and β and γ regulatory units that jointly make a heterotrimeric functional enzyme. The multiple AMPK subunit isoforms encoded by distinct genes are expressed in different tissues such as the liver, brain, and skeletal muscle and therefore have a tissue-specific distinct distribution.50,51 Among the seven isoforms of AMPK, the α2 and β2 isoforms are significantly expressed in the heart. Each subunit isoform’s differential distributions provide a tissue-selective regulation of the AMPK activity.52
AMPK activation occurs by an increase in the concentration of AMP and its binding to the γ subunit, which induces a conformational alteration in the AMPK structure, and allosterically actuating assembly to the α catalytic subunit, triggers phosphorylation of the Thr172 residue by upstream AMPK kinases, and prevents the effect of protein phosphatase 2C from dephosphorylating Thr172.53
Some clinically used drugs pharmacologically activate AMPK in conventional medicine, derived from natural sources in traditional medicines, food, or beverages.54 5-Aminoimidazole-4-carboxamide riboside (AICA riboside), an adenosine analog, mimics AMP’s effects on the AMPK complex and activates AMPK in most cells.55 Metformin, phenformin, and glargine increase the cellular ADP: ATP ratio and activate AMPK by inhibiting Complex I of the respiratory chain.56 The anti-diabetic medicines of thiazolidinediones class activate AMPK both by a humoral, adiponectin-dependent procedure and through a cell-autonomous, adiponectin-independent mechanism.54 2-Deoxyglucose (2DG), as a repressor of glycolysis, activates AMPK partially by restoring ATP, causing an increase in ADP: ATP due to its phosphorylation by hexokinase.56 A-769662, a thienopyridine compound, mimics AMP’s effects on the AMPK system, i.e., allosteric activation and protection against Thr-172 dephosphorylation.57 The natural ligand of salicylate binds at a position that interferes with that used by A-769662. It is considered a direct activator of AMPK with less potency than A-769662.58 Phenobarbital,59 plant products like resveratrol,60 epigallocatechin-3-gallate,61 curcumin,62 garlic oil,63 berberine,64 hispidulin65 can also activate AMPK. PT1, a small molecule activator of AMPK, promotes ACC phosphorylation without altering the AMP: ATP ratio.66 Inhibition of AMP-metabolizing enzymes like phosphodiesterases, by inhibitors like sildenafil, may also be considered as AMPK activators.67
Accumulating evidence from in vitro and in vivo experiments indicates the involvement of AMPK as a negative regulator of immune/inflammatory responses, and shaping the cell’s metabolic status at sites of inflammation may serve as a novel and promising therapeutic approach in the pathophysiology of several immune-mediated inflammatory diseases. The anti-inflammatory effects of AMPK activators have been shown to confer benefits in colitis,6,68 hepatitis and liver disturbances,69,70 autoimmune encephalomyelitis,71 osteoarthritis,72 endotoxemia,73-75 MI,76 angiogenesis,77 acute kidney injuries,78 and mouse lung injury model.79
AMPK is consistent with the suppressed production of pro-inflammatory mediators and the promotion of anti-inflammatory cytokines.80 Some of the main molecular anti-inflammatory mechanisms of AMPK were reviewed by Salt and Palmer81 in 2012 and shown in Figure 2, which include inhibition of cytokine-stimulated iNOS (inducible NO synthase) protein expression and inhibition of NF-kB signaling,82 inhibition of MAPK pathways,83 modulations of reactive oxygen species (ROS) synthesis,84,85 inhibition of JAK-STAT signaling,86 prevention of leukocyte infiltration,87 regulation of cytokine synthesis88 and regulation of lipid metabolism by maintaining fatty acid oxidation in macrophages to limit inflammation.89
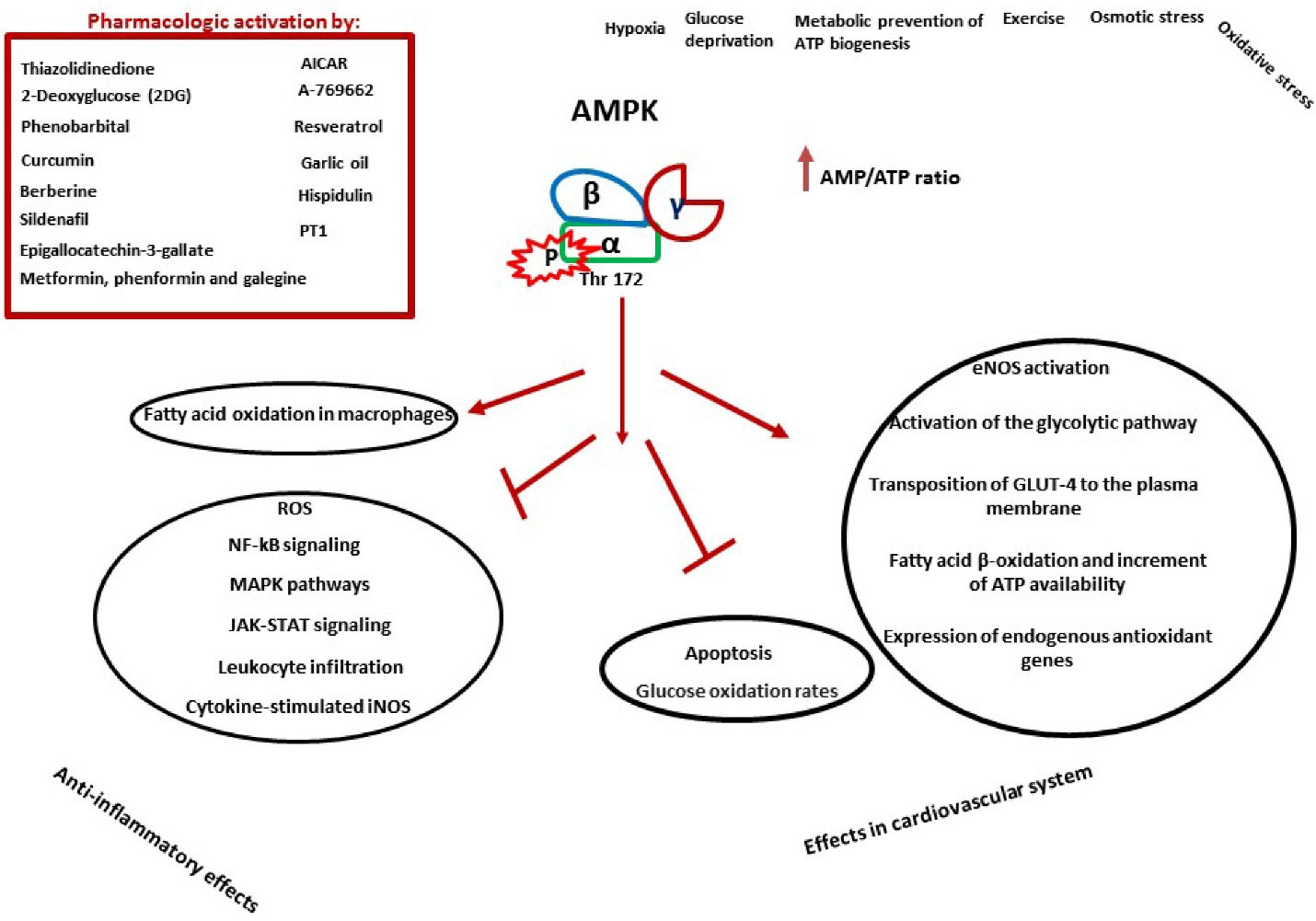
Figure 2.
AMPK is phosphorylated and activated by various agents. These factors can be energy depleting conditions (such as hypoxia, metabolic prevention of ATP biogenesis, etc.) or pharmacological agents (for example: metformin, A-769662, etc.). After phosphorylation in threonine 172 α subunit, different pathways are activated. AMPK activation demonstrate anti-inflammatory effects and also ambivalent effects on the cardiovascular system. Some of the main anti-inflammatory mechanisms of AMPK are through inhibition of NF-κB signaling, MAPK pathways, JAK-STAT signaling, leukocyte infiltration, cytokine-stimulated iNOS and increased fatty acid oxidation in macrophages. In the cardiovascular system, activation of AMPK can reduce glucose oxidation and apoptosis, on the other hand, it can increase fatty acid oxidation, GLUT-4 transport to the plasma membrane, glycolysis, eNOS activation and expression of antioxidant genes. AICAR: 5-Aminoimidazole-4-carboxamide riboside; PT1: AMPK activator; ROS: reactive oxygen species; GLUT-4: glucose transporter 4; eNOS: endothelial isoform of NO synthase. →: Stimulation/Increase; ┤: Inhibition/Decrease.
Figure 2.
AMPK is phosphorylated and activated by various agents. These factors can be energy depleting conditions (such as hypoxia, metabolic prevention of ATP biogenesis, etc.) or pharmacological agents (for example: metformin, A-769662, etc.). After phosphorylation in threonine 172 α subunit, different pathways are activated. AMPK activation demonstrate anti-inflammatory effects and also ambivalent effects on the cardiovascular system. Some of the main anti-inflammatory mechanisms of AMPK are through inhibition of NF-κB signaling, MAPK pathways, JAK-STAT signaling, leukocyte infiltration, cytokine-stimulated iNOS and increased fatty acid oxidation in macrophages. In the cardiovascular system, activation of AMPK can reduce glucose oxidation and apoptosis, on the other hand, it can increase fatty acid oxidation, GLUT-4 transport to the plasma membrane, glycolysis, eNOS activation and expression of antioxidant genes. AICAR: 5-Aminoimidazole-4-carboxamide riboside; PT1: AMPK activator; ROS: reactive oxygen species; GLUT-4: glucose transporter 4; eNOS: endothelial isoform of NO synthase. →: Stimulation/Increase; ┤: Inhibition/Decrease.
AMPK and CVD
AMPK has various implications of great importance in the cardiovascular system. While AMPK signaling has a particular physiological role in the heart cells like other tissues, its importance relies on its activation by stress conditions like an extra hemodynamic burden, myocardial ischemia, and hypoxia. Under the mentioned conditions, the energy metabolism role of AMPK is to maintain sufficient ATP levels by improving the uptake of glucose by transposition of glucose transporter 4 (GLUT-4) into the cardiomyocytes,90 activation of the glycolytic pathway by phosphorylating phosphofructokinase-2, augmentation of fatty acid β-oxidation, and increasing ATP availability.91-93
AMPK induces endothelial isoform of NO synthase (eNOS) activation,94 which leads to the generation and release of NO from the endothelium. NO as an endothelial protective agent play a role by the promotion of vascular smooth muscle relaxation and proliferation, inhibition of leukocyte adherence and migration, platelet aggregation, and production of adhesion molecules.95
Different studies reviewed by Shirwany and Zou93 in 2010 suggest a complicated relation between the AMPK cascade and the redox ratio in cardiovascular homeostasis. This correlation emerged to be free from its assumed function energy-conserving pathways in the cell. AMPK regulates the antioxidant system by expressing endogenous antioxidant genes and repressing oxidants’ synthesis into cell survival.93
Plenty of studies have been monitoring the relation of AMPK with the different pathophysiological status of the cardiovascular system. They indicated that AMPK can modulate the cardiac hypertrophy procedure,96 potentiate preconditioning effect in the ischemic heart,97 involves in the pathogenesis of the Wolf-Parkinson-White syndrome in case of mutations in the γ2 subunit of AMPK,98 plays as a prominent constitute in angiogenesis and neointimal hyperplasia and also unclarified traces of this enzyme was observed in atherosclerosis development.99
AMPK, through regulating ATP synthesis and consumption controls energy metabolism, is assumed to be a promising target for heart protection ischemic injuries.100
In the ischemic condition of the myocardium, increased rates of fatty acid oxidation consequently inhibit glucose oxidation. Also, accelerated glycolysis during ischemia increases lactate production and intracellular acidosis by promoting calcium overload during the reperfusion period. As an essential regulator of fatty acid oxidation, malonyl-CoA prevents the uptake of fatty acids into the mitochondria. AMPK phosphorylates and inhibits acetyl-CoA carboxylase (ACC), suppressing the synthesis of malonyl-CoA. Furthermore, it phosphorylates and activates malonyl-CoA decarboxylase (MCD), increasing the degradation of malonyl-CoA levels, resulting in a decreased rate of glucose oxidation.101,102
Fatty acid oxidation of AMPK may attenuate glucose oxidation, leading to acidosis due to increased lactate and proton production. This influence cardiac efficiency and result in the severity of ischemic injuries. In this case, the prohibition of the ischemic-induced activation of AMPK and suppressing the downstream decline in the malonyl-CoA ratio support the notion that it may be a novel and beneficial therapeutic strategy to decrease ischemic heart disease outcomes.102
On the other side, there is a critical role of AMPK activation in glucose uptake and ATP production, especially in the energy deprivation status of ischemia, through translocation of the glucose transporter 4 from intracellular compartments the cell surface.103 Furthermore, apoptotic procedure induced by ischemia and infarction increased in AMPK-deleted mice,104,105 expressing the importance of AMPK as a double-edged sword.
AMPK and TLR4 relation
The correlation between AMPK and TLR4 is a novel research topic, with various unknown aspects; different studies have mentioned this and tried to determine how the relationship exists. A study showed that aerobic exercise could improve insulin resistance, blood glucose level, and lipid profile in diabetic mice. The authors attributed the beneficial effects to the TLR4 mediated ERK/AMPK signaling pathway in this study. Reporting that, the upregulation of TLR4 expression level increases ERK and AMPK activity.106 Another study showed that dihydroquercetin reduced inflammation in LPS-induced endotoxemia through enhancement of AMPK phosphorylation and downregulation of TLR4 expression.75 Another link of AMPK and TLR4 were documented in hypothalamic pharmacological activation of AMPK (hyp-AMPK) in LPS-treated mice. It was reported that hyp-AMPK activation suppressed the effects of LPS on the hyp-AMPK phosphorylation, liver PEPCK expression, and glucose generation in a TLR4-dependent manner because none of these changes were affected in TLR4-mutant mice. These findings demonstrated the relation between hypothalamic AMPK dephosphorylation and TLR4 protein in hypoglycemia evoked by LPS. Although TLR4 expression in the hypothalamus was not modified in LPS-treated mice, on the contrary, transforming growth factor b-activated kinase 1 (TAK1) phosphorylation and TLR4/MYD88 association were increased.12 TLR and AMPK relation in reducing adipogenesis, adipose inflammation, and promoting energy expenditure recently was confirmed in a mice model of obesity by modulating SIRT1 (Sirtuin 1, an enzyme function as intracellular regulatory proteins), TNF-α and IL-6 associated metabolic and inflammatory pathways.107
Despite numerous references to the anti-inflammatory role of AMPK, the pro-inflammatory impress of AMPK in TLR4 cascade was mentioned by Kim et al108 in 2012. They demonstrated that AMPK-α1 has an essential upstream kinases role in stimulating pro-inflammatory signals via the activation of TAK1. The deficiency of AMPK-α1 or inhibition of AMPK-α1 activity by compound C consequence in the dramatic decrease in TAK1 activity, subsequent blocking of downstream signaling cascades, and the expression of NF-kB-dependent genes in response to LPS stimulation, pointing out to an AMPK-α1-TAK1-NF-kB axis in TLR4-mediated signaling. One of the valuable studies that can help clarify the relationship between TLR4 and AMPK was the research done by Kim et al.109 On the evaluation of anti-inflammatory effects of Andrographolide, a well-known labdane diterpenoid of Andrographis paniculata. Andrographolide significantly reduced LPS-induced pro-inflammatory cytokines by suppressing NF-κB, MAPK, and their upstream signaling pathways through activating the AMPK. They showed that activation of AMPK could inhibit ERK/JNK/p38 pathway. Simultaneously, directly interact with TAK1, inhibit TAK1-dependent MAPK signaling cascade, and subsequently inhibit NF-kB induced pro-inflammatory cytokine genes.
The other link that turned light in determining TLR4 and AMPK relation reported by Yuk et al.39 They showed that activation of NF-κB via LPS-induced TLR signaling evoked SHP transcription via a CaMKKβ–AMPK–USP1-dependent signaling pathway that eventually suppressed NF-κB in a negative feedback loop. It is demonstrated that AMPK, via the Nrf2/antioxidant responsive element (Nrf2/ARE) signaling pathway, stimulated the heme oxygenase (HO)-1 system, which diminished cellular stress and inhibited TLR signaling.110 Severe inflammation have been demonstrated in HO-1 knockout mice and in a human case of genetic HO-1 deficiency, which showed the significant anti-inflammatory role of the HO-1/CO system.111 Upon AMPK activation, it stimulates SIRT1, Peroxisome proliferator-activated receptor-γ coactivator (PGC)-1α, Forkhead transcription factor (FoxO), and p53, which could attenuate NF-κB activation. AMPK is a master controller of PGC1α, a transcriptional coactivator described as a master regulator of mitochondrial gene expression, including oxidative phosphorylation and ROS detoxification, and is associated with the pathogenesis of the CVD. PGC-1α and NF-κB are mutually regulated during inflammation, where oxidative stress plays an essential role.112 FoxOs are phosphorylated and activated by AMPK. Many investigators have demonstrated that FoxO plays a crucial role in inducing various downstream genes involved in oxidative stress response and suppressing ROS. FoxO6 suppresses pro-inflammatory gene up-regulation by NF-κB, and thus reducing oxidative stress.113
The various mechanisms in the TLR4-inflammatory pathway which can undergo modulation by AMPK are demonstrated in Figure 3. However, it should be noted that the role of AMPK in inflammatory pathways is complicated and may be conflicting depended on specific cell types.
Figure 3.
AMPK can ameliorate inflammatory responses via various direct and indirect anti-inflammatory signaling pathways. Engagement of TLRs by DAMPs activates inflammatory responses and upon TLR4 stimulation, the activation of AMPK can be simultaneously induced by LKB1 and Ca2+-calmodulin-dependent protein kinase. Activation of AMPK can inhibit ERK/JNK/p38 pathway. Simultaneously, directly interact with TAK1 and inhibit TAK1-dependent MAPK signaling cascade and subsequently inhibit NF-κB-induced pro-inflammatory cytokine genes. ↓, activation; ⊥, inhibition. AMPK, AMP-activated kinase; AP-1, activator protein 1; CaMKKβ, Ca2+/calmodulin-dependent protein kinase kinase-β; FoxO, Forkhead transcription factor; HO-1/CO, heme oxygenase-1/carbon monoxide; PGC-1α, Peroxisome proliferator-activated receptor-γ coactivator; SHP, small heterodimer partner; SIRT1, Sirtuin 1; TLR, Toll-like receptor.
Figure 3.
AMPK can ameliorate inflammatory responses via various direct and indirect anti-inflammatory signaling pathways. Engagement of TLRs by DAMPs activates inflammatory responses and upon TLR4 stimulation, the activation of AMPK can be simultaneously induced by LKB1 and Ca2+-calmodulin-dependent protein kinase. Activation of AMPK can inhibit ERK/JNK/p38 pathway. Simultaneously, directly interact with TAK1 and inhibit TAK1-dependent MAPK signaling cascade and subsequently inhibit NF-κB-induced pro-inflammatory cytokine genes. ↓, activation; ⊥, inhibition. AMPK, AMP-activated kinase; AP-1, activator protein 1; CaMKKβ, Ca2+/calmodulin-dependent protein kinase kinase-β; FoxO, Forkhead transcription factor; HO-1/CO, heme oxygenase-1/carbon monoxide; PGC-1α, Peroxisome proliferator-activated receptor-γ coactivator; SHP, small heterodimer partner; SIRT1, Sirtuin 1; TLR, Toll-like receptor.
AMPK and TLR 4 relation in the cardiovascular system
In 2012, it was confirmed that acute treatment with metformin improves ECG pattern and cardiac function following isoproterenol-induced MI.114 After this study, it was indicated that short-term administration of metformin in MI, particularly in an AMPK activating dose, profoundly suppressed post-MI remodeling and pro-inflammatory reactions, as demonstrated by a decline in the myeloperoxidase activity in the myocardium and a reduction in the TNF-α and IL-6 content in the heart tissue and serum. These results were correlated with the suppression of the mRNA level of TLR4 in the cardiac tissue and the diminution of the protein level of MyD88, which were elevated in the myocardium following isoproterenol-induced MI.13 This finding of AMPK activation by metformin and the subsequent inhibition of TLR4 expression and activity led to the hypothesis that there may be a link between AMPK and TLRs. The importance of AMPK as a cardioprotective agent against ischemic injury of the heart also was suggested in a model of deficiency in TLR4 signaling. It was shown that in regional ischemia induced in wild type c3H/HeN and Tlr-4 mutated C3H/HeJ mice, elimination of Tlr-4 had a cardio-protective outcome on ischemic damages due to activation of AMPK and ERK signaling pathways. It was explanatory that TLR4 is negatively correlated to AMPK activation in the heart following ischemia-reperfusion.14 Also, Fang et al115 indicated that the immune reaction processes are interceded by the TLR4 signaling cascade (MyD88-dependent pathway and TRIF-dependent pathway) interfacing with other pathways (PI3K/Akt and AMPK, ERK signaling pathway). Investigation on modulating fatty acid metabolism after diabetes declared that although diabetes increased AMPK phosphorylation in both non-obese diabetic mice and TLR4-deficient non-obese diabetic mice, however, TLR4-deficient group demonstrated a higher level of AMPK and acetyl-CoA carboxylase phosphorylation. Thereby, it was speculated that TLR4 deletion might boost the upregulation of cardiac fatty acids metabolism via AMPK activation following diabetes and indicate a potentially significant relation of TLR4 and AMPK in the progression of diabetic cardiomyopathy and dysfunction.116
A study showed that high-mobility groupbox 1 (HMGB1) plays a pathological role in doxorubicin-induced heart failure via TLR4. They showed that inhibition of HMGB1 exerts cardioprotective effects by increasing AMPK protein level and preventing cardiomyocyte apoptosis.117 Concerning this, it was reported that TLR4 engagement inhibits AMPK activation through an HMGB1 protein-dependent mechanism in LPS-induced acute lung injury.118
Recently, in one of our published studies, we showed that metformin, as an AMPK activator, protects the lung tissue from LPS-induced acute injury through activation of AMPK and suppression of TLR4.20 This pointed to the fact that the linkage of TLR4 and AMPK is not limited to cardiac tissue. Therefore the beneficial effects of metformin in cardiac activity in septic crisis may be due to other body reflexes, including anti-inflammatory roles in other organs such as the lung. To examine this hypothesis, we performed the isolated heart modeling of sepsis by using LPS. What was acquired rejected this supposition, because again, metformin suppressed TLR4 expression via the AMPK-dependent pathway. It pointed to the significance of TLR4-involved local immune reactions in the LPS-induced myocardial dysfunction. It inferred a clear connection between AMPK and TLR4 even far from the systemic circuit and circulatory immunity.22 Using metformin in sepsis induced myocardial dysfunction, reduced TLR4 gene expression and decreased the protein content of MYD88 and TNF-α level in the heart. Interestingly, these effects again were AMPK-dependent, and inhibition of AMPK by compound C reversed the influence of metformin in this endotoxemic model.119
A study by Sun et al120 demonstrated that vaccariae hypaphorine (VH), the main active compound of the Vaccaria segetalis, reduced LPS-induced inflammatory cytokine production in human endothelial EA·hy926 cells. In this study, pre-treatment with AICAR and A769662, AMPK activators, decreased TLR4 and increased PPARγ protein levels in LPS-treated cells. They concluded that this anti-inflammatory effect is attributed to the inhibition of TLR4 and activation of PPARγ, which is dependent on the AMPK signaling pathway. Another study investigating diet-induced obesity on cardiac inflammatory responses showed that a high-fat diet increased the inflammatory responses and decreased glucose metabolism in the heart via suppression of AMPK activity. In this study, acute lipid infusion increased circulating fatty acids. As a result, cardiac inflammation happens by activating the TLR4 signaling pathway.121
In summary, considering the studies mentioned above, it can be said that there is a relationship between APMK and TLR4. In most cases, this relationship is negative feedback.
Expert opinion
Inflammation is related to many diseases, together with cardiovascular complications. Innate immunity has ruled studies in CVDs. However, the significance of innate immunity results in new research regions. It might bring about novel therapeutic targets for treating inflammatory diseases. AMPK is an essential target given its co-regulatory function concerning metabolism and inflammation.
On the other hand, broad studies have been performed on TLR modulators and their therapeutic indication in several cardiac-related disorders. Albeit, the interaction of these two critical factors in inflammatory processes needs to be clarified. The significant limitation currently facing these studies is the lack of knowledge about how effective these pathways will be. This is likely due to the complexity of cell signaling in inflammatory conditions, especially the bilateral effects of TLR’s role in either improving immune-related diseases or exacerbating them. Furthermore, depending on the context and stage of the disease, several inflammatory pathways are relevant, requiring further study in this area. Despite the various studies related to perspectives of TLR modulation,122 though the lack of clear understanding of the role of TLR receptors in the progression of the specific disease is one of the main hampering reasons.
Nevertheless, a sensible therapeutic approach must be considered, which will not over-activate or -inhibit the immune defense role. The practical appearance of new immune modulator drugs by interfering AMPK pathway requires a more in-depth identification and understanding of the function and localization of different TLR receptors and various mediators in AMPK and TLR signaling pathways.
Future studies might examine whether therapeutic interventions through AMPK related approaches can improve inflammatory disorders in immune-based disease. Furthermore, as the interference of inflammation in vast ranges of diseases spanning from organ-dependent inflammation like a CVD to systemic conditions such as autoimmune disorders and cancers; thereby any attempt to identify novel therapeutic targets within this system seems justified.
Another use for these novel medicines will be in cardio-inflammatory diseases, which this review article, and chronic disorders with subsequent cardiovascular events such as diabetes, atherosclerosis, and hypertension. Since the footprint of inflammatory mechanisms is confirmed in the progression of all these high prevalence pathologic conditions, more profound exploration into this treatment approach can ultimately promote the development of the life sciences.
Moreover, TLRs’ potential property in distinguishing PAMPs of diverse origin and commencing of pro-inflammatory response during various inflammatory conditions. During indication for sepsis, many cases of effectiveness in a completely different type of disease were reported, which lead the researches on their impact and indication in various conditions such as influenza-associated acute lung injury, hepatitis B, human papillomavirus, metastatic melanoma, non-small cell lung cancer and even controlling mammalian reproduction and fertilization processes.123
In our opinion, novel AMPK-related TLR modulators will find application in treating different immune-mediated inflammatory and non-inflammatory disorders, especially inflammatory cardiac disease, and present an option that will be widely used in clinical practice in the future.
Conclusion
This review elaborates on the possible cross-talk between TLR4 and AMPK signaling in CVDs. In summary, the appropriate inflammatory response induced by TLRs is required to function both innate and adaptive immune responses to defend against damage and tissue repair. However, its inordinate signaling may be devastating and can result in cardiac disorders. Among all TLRs, TLR4 has been extensively investigated for its critical role in different myocardial inflammatory conditions. The results of several studies are explanatory of a theory that there may be a relationship between TLR4 and AMPK signaling in various inflammatory conditions. AMPK, a serine/threonine kinase, acts as a cellular energy sensor and is activated due to ATP depletion. Although AMPK signaling has a particular physiological role in the heart cells like other tissues, its importance is accentuated in stressor conditions such as myocardial ischemia. Most of the evidence from previous research highlights the anti-inflammatory benefits of the AMPK activators that point out a novel and promising therapeutic strategy in the pathophysiology of several immune-mediated inflammatory diseases. Finally, it should be noted that the correlation between AMPK and TLRs is a novel and investigational subject, with various unknown aspects, which need further research and attention.
Acknowledgments
The authors duly acknowledge Vice Chancellor for Research of Tabriz University of Medical Sciences, Tabriz, Iran for their support.
Author Contributions
Conceptualization: Haleh Vaez.
Data Creation: Haleh Vaez, Hamid Soraya.
Investigation: Haleh Vaez, Hamid Soraya.
Methodology: Haleh Vaez, Alireza Garjani.
Project administration: Haleh Vaez.
Supervision: Alireza Garjani.
Ethical Issues
Not applicable.
Conflict of Interest
The authors declare that they have no competing interests.
References
- Amarante-Mendes GP, Adjemian S, Branco LM, Zanetti LC, Weinlich R, Bortoluci KR. Pattern recognition receptors and the host cell death molecular machinery. Front Immunol 2018; 9:2379. doi: 10.3389/fimmu.2018.02379 [Crossref] [ Google Scholar]
- Vénéreau E, Ceriotti C, Bianchi ME. DAMPs from cell death to new life. Front Immunol 2015; 6:422. doi: 10.3389/fimmu.2015.00422 [Crossref] [ Google Scholar]
- Rameshrad M, Soraya H, Maleki-Dizaji N, Vaez H, Garjani A. A-769662, a direct AMPK activator, attenuates lipopolysaccharide-induced acute heart and lung inflammation in rats. Mol Med Rep 2016; 13(3):2843-9. doi: 10.3892/mmr.2016.4821 [Crossref] [ Google Scholar]
- Fullerton MD, Steinberg GR, Schertzer JD. Immunometabolism of AMPK in insulin resistance and atherosclerosis. Mol Cell Endocrinol 2013; 366(2):224-34. doi: 10.1016/j.mce.2012.02.004 [Crossref] [ Google Scholar]
- O’Neill LA, Hardie DG. Metabolism of inflammation limited by AMPK and pseudo-starvation. Nature 2013; 493(7432):346-55. doi: 10.1038/nature11862 [Crossref] [ Google Scholar]
- Bai A, Ma AG, Yong M, Weiss CR, Ma Y, Guan Q. AMPK agonist downregulates innate and adaptive immune responses in TNBS-induced murine acute and relapsing colitis. BiochemPharmacol 2010; 80(11):1708-17. doi: 10.1016/j.bcp.2010.08.009 [Crossref] [ Google Scholar]
- Myerburg MM, King JD Jr, Oyster NM, Fitch AC, Magill A, Baty CJ. AMPK agonists ameliorate sodium and fluid transport and inflammation in cystic fibrosis airway epithelial cells. Am J Respir Cell Mol Biol 2010; 42(6):676-84. doi: 10.1165/2009-0147oc [Crossref] [ Google Scholar]
- Abrams J. Abrams JClinical practiceChronic stable angina. N Engl J Med 2005; 352(24):2524-33. doi: 10.1056/NEJMcp042317 [Crossref] [ Google Scholar]
- Southern BD, Scheraga RG, Olman MA. Impaired AMPK activity drives age-associated acute lung injury after hemorrhage. Am J Respir Cell Mol Biol 2017; 56(5):553-5. doi: 10.1165/rcmb.2017-0023ED [Crossref] [ Google Scholar]
- Park DW, Jiang S, Tadie JM, Stigler WS, Gao Y, Deshane J. Activation of AMPK enhances neutrophil chemotaxis and bacterial killing. Mol Med 2013; 19(1):387-98. doi: 10.2119/molmed.2013.00065 [Crossref] [ Google Scholar]
- Zhao X, Zmijewski JW, Lorne E, Liu G, Park YJ, Tsuruta Y. Activation of AMPK attenuates neutrophil proinflammatory activity and decreases the severity of acute lung injury. Am J Physiol Lung Cell Mol Physiol 2008; 295(3):L497-504. doi: 10.1152/ajplung.90210.2008 [Crossref] [ Google Scholar]
- Santos GA, Moura RF, Vitorino DC, Roman EA, Torsoni AS, Velloso LA. Hypothalamic AMPK activation blocks lipopolysaccharide inhibition of glucose production in mice liver. Mol Cell Endocrinol 2013; 381(1-2):88-96. doi: 10.1016/j.mce.2013.07.018 [Crossref] [ Google Scholar]
- Soraya H, Farajnia S, Khani S, Rameshrad M, Khorrami A, Banani A. Short-term treatment with metformin suppresses toll like receptors (TLRs) activity in isoproterenol-induced myocardial infarction in rat: are AMPK and TLRs connected?. Int Immunopharmacol 2012; 14(4):785-91. doi: 10.1016/j.intimp.2012.10.014 [Crossref] [ Google Scholar]
- Zhao P, Wang J, He L, Ma H, Zhang X, Zhu X. Deficiency in TLR4 signal transduction ameliorates cardiac injury and cardiomyocyte contractile dysfunction during ischemia. J Cell Mol Med 2009; 13(8a):1513-25. doi: 10.1111/j.1582-4934.2009.00798.x [Crossref] [ Google Scholar]
- Chao W. Toll-like receptor signaling: a critical modulator of cell survival and ischemic injury in the heart. Am J Physiol Heart Circ Physiol 2009; 296(1):H1-12. doi: 10.1152/ajpheart.00995.2008 [Crossref] [ Google Scholar]
- Lemaitre B, Nicolas E, Michaut L, Reichhart JM, Hoffmann JA. The dorsoventral regulatory gene cassette spätzle/Toll/cactus controls the potent antifungal response in Drosophila adults. Cell 1996; 86(6):973-83. doi: 10.1016/s0092-8674(00)80172-5 [Crossref] [ Google Scholar]
- Medzhitov R, Preston-Hurlburt P, Janeway CA Jr. A human homologue of the Drosophila Toll protein signals activation of adaptive immunity. Nature 1997; 388(6640):394-7. doi: 10.1038/41131 [Crossref] [ Google Scholar]
- Blasius AL, Beutler B. Intracellular toll-like receptors. Immunity 2010; 32(3):305-15. doi: 10.1016/j.immuni.2010.03.012 [Crossref] [ Google Scholar]
- Mitchell JA, Ryffel B, Quesniaux VF, Cartwright N, Paul-Clark M. Role of pattern-recognition receptors in cardiovascular health and disease. Biochem Soc Trans 2007; 35(Pt 6):1449-52. doi: 10.1042/bst0351449 [Crossref] [ Google Scholar]
- Vaez H, Najafi M, Seyed Toutounchi N, Barar J, Barzegari A, Garjani A. Metformin alleviates lipopolysaccharide-induced acute lung injury through suppressing toll-like receptor 4 signaling. Iran J Allergy Asthma Immunol 2016; 15(6):498-507. [ Google Scholar]
- Rameshrad M, Maleki-Dizaji N, Vaez H, Soraya H, Nakhlband A, Garjani A. Lipopolysaccharide induced activation of toll like receptor 4 in isolated rat heart suggests a local immune response in myocardium. Iran J Immunol 2015; 12(2):104-16. [ Google Scholar]
- Vaez H, Najafi M, Rameshrad M, Seyed Toutounchi N, Garjani M, Barar J. AMPK activation by metformin inhibits local innate immune responses in the isolated rat heart by suppression of TLR 4-related pathway. Int Immunopharmacol 2016; 40:501-7. doi: 10.1016/j.intimp.2016.10.002 [Crossref] [ Google Scholar]
- Soraya H, Clanachan AS, Rameshrad M, Maleki-Dizaji N, Ghazi-Khansari M, Garjani A. Chronic treatment with metformin suppresses toll-like receptor 4 signaling and attenuates left ventricular dysfunction following myocardial infarction. Eur J Pharmacol 2014; 737:77-84. doi: 10.1016/j.ejphar.2014.05.003 [Crossref] [ Google Scholar]
- Ding HS, Yang J, Chen P, Yang J, Bo SQ, Ding JW. The HMGB1-TLR4 axis contributes to myocardial ischemia/reperfusion injury via regulation of cardiomyocyte apoptosis. Gene 2013; 527(1):389-93. doi: 10.1016/j.gene.2013.05.041 [Crossref] [ Google Scholar]
- Liu L, Wang Y, Cao ZY, Wang MM, Liu XM, Gao T. Up-regulated TLR4 in cardiomyocytes exacerbates heart failure after long-term myocardial infarction. J Cell Mol Med 2015; 19(12):2728-40. doi: 10.1111/jcmm.12659 [Crossref] [ Google Scholar]
- Zeng Q, Song R, Ao L, Weyant MJ, Lee J, Xu D. Notch1 promotes the pro-osteogenic response of human aortic valve interstitial cells via modulation of ERK1/2 and nuclear factor-κB activation. ArteriosclerThrombVasc Biol 2013; 33(7):1580-90. doi: 10.1161/atvbaha.112.300912 [Crossref] [ Google Scholar]
- Bagheri B, Sohrabi B, Movassaghpour AA, Mashayekhi S, Garjani A, Shokri M. Hydrocortisone reduces toll-like receptor 4 expression on peripheral CD14+ monocytes in patients undergoing percutaneous coronary intervention. Iran Biomed J 2014; 18(2):76-81. doi: 10.6091/ibj.1275.2013 [Crossref] [ Google Scholar]
- Satoh S, Yada R, Inoue H, Omura S, Ejima E, Mori T. Toll-like receptor-4 is upregulated in plaque debris of patients with acute coronary syndrome more than toll-like receptor-2. Heart Vessels 2016; 31(1):1-5. doi: 10.1007/s00380-014-0565-9 [Crossref] [ Google Scholar]
- Park BS, Lee JO. Recognition of lipopolysaccharide pattern by TLR4 complexes. Exp Mol Med 2013; 45(12):e66. doi: 10.1038/emm.2013.97 [Crossref] [ Google Scholar]
- Tumurkhuu G, Dagvadorj J, Jones HD, Chen S, Shimada K, Crother TR. Alternatively spliced myeloid differentiation protein-2 inhibits TLR4-mediated lung inflammation. J Immunol 2015; 194(4):1686-94. doi: 10.4049/jimmunol.1402123 [Crossref] [ Google Scholar]
- Wright SD, Ramos RA, Tobias PS, Ulevitch RJ, Mathison JC. CD14, a receptor for complexes of lipopolysaccharide (LPS) and LPS binding protein. Science 1990; 249(4975):1431-3. doi: 10.1126/science.1698311 [Crossref] [ Google Scholar]
- Kopp F, Kupsch S, Schromm AB. Lipopolysaccharide-binding protein is bound and internalized by host cells and colocalizes with LPS in the cytoplasm: implications for a role of LBP in intracellular LPS-signaling. BiochimBiophys Acta 2016; 1863(4):660-72. doi: 10.1016/j.bbamcr.2016.01.015 [Crossref] [ Google Scholar]
- El-Zayat SR, Sibaii H, Mannaa FA. Toll-like receptors activation, signaling, and targeting: an overview. Bull Natl Res Cent 2019; 43(1):187. doi: 10.1186/s42269-019-0227-2 [Crossref] [ Google Scholar]
- Hinz M, Scheidereit C. The IκB kinase complex in NF-κB regulation and beyond. EMBO Rep 2014; 15(1):46-61. doi: 10.1002/embr.201337983 [Crossref] [ Google Scholar]
- Barton GM, Medzhitov R. Toll-like receptor signaling pathways. Science 2003; 300(5625):1524-5. doi: 10.1126/science.1085536 [Crossref] [ Google Scholar]
- Farrugia M, Baron B. The role of toll-like receptors in autoimmune diseases through failure of the self-recognition mechanism. Int J Inflam 2017; 2017:8391230. doi: 10.1155/2017/8391230 [Crossref] [ Google Scholar]
- Liew FY, Xu D, Brint EK, O’Neill LA. Negative regulation of toll-like receptor-mediated immune responses. Nat Rev Immunol 2005; 5(6):446-58. doi: 10.1038/nri1630 [Crossref] [ Google Scholar]
- Guven-Maiorov E, Keskin O, Gursoy A, Nussinov R. A structural view of negative regulation of the toll-like receptor-mediated inflammatory pathway. Biophys J 2015; 109(6):1214-26. doi: 10.1016/j.bpj.2015.06.048 [Crossref] [ Google Scholar]
- Yuk JM, Shin DM, Lee HM, Kim JJ, Kim SW, Jin HS. The orphan nuclear receptor SHP acts as a negative regulator in inflammatory signaling triggered by toll-like receptors. Nat Immunol 2011; 12(8):742-51. doi: 10.1038/ni.2064 [Crossref] [ Google Scholar]
- Yu L, Feng Z. The role of toll-like receptor signaling in the progression of heart failure. Mediators Inflamm 2018; 2018:9874109. doi: 10.1155/2018/9874109 [Crossref] [ Google Scholar]
- Wang E, Feng Y, Zhang M, Zou L, Li Y, Buys ES. Toll-like receptor 4 signaling confers cardiac protection against ischemic injury via inducible nitric oxide synthase- and soluble guanylate cyclase-dependent mechanisms. Anesthesiology 2011; 114(3):603-13. doi: 10.1097/ALN.0b013e31820a4d5b [Crossref] [ Google Scholar]
- Monnerat-Cahli G, Alonso H, Gallego M, Alarcón ML, Bassani RA, Casis O. Toll-like receptor 4 activation promotes cardiac arrhythmias by decreasing the transient outward potassium current (Ito) through an IRF3-dependent and MyD88-independent pathway. J Mol Cell Cardiol 2014; 76:116-25. doi: 10.1016/j.yjmcc.2014.08.012 [Crossref] [ Google Scholar]
- Biancardi VC, Bomfim GF, Reis WL, Al-Gassimi S, Nunes KP. The interplay between angiotensin II, TLR4 and hypertension. Pharmacol Res 2017; 120:88-96. doi: 10.1016/j.phrs.2017.03.017 [Crossref] [ Google Scholar]
- Campbell MT, Hile KL, Zhang H, Asanuma H, Vanderbrink BA, Rink RR. Toll-like receptor 4: a novel signaling pathway during renal fibrogenesis. J Surg Res 2011; 168(1):e61-9. doi: 10.1016/j.jss.2009.09.053 [Crossref] [ Google Scholar]
- Feng Q, Liu D, Lu Y, Liu Z. The interplay of renin-angiotensin system and toll-like receptor 4 in the inflammation of diabetic nephropathy. J Immunol Res 2020; 2020:6193407. doi: 10.1155/2020/6193407 [Crossref] [ Google Scholar]
- Zhang Y, Peng W, Ao X, Dai H, Yuan L, Huang X. TAK-242, a toll-like receptor 4 antagonist, protects against aldosterone-induced cardiac and renal injury. PLoS One 2015; 10(11):e0142456. doi: 10.1371/journal.pone.0142456 [Crossref] [ Google Scholar]
- Avlas O, Bragg A, Fuks A, Nicholson JD, Farkash A, Porat E. TLR4 expression is associated with left ventricular dysfunction in patients undergoing coronary artery bypass surgery. PLoS One 2015; 10(6):e0120175. doi: 10.1371/journal.pone.0120175 [Crossref] [ Google Scholar]
- Yang Y, Lv J, Jiang S, Ma Z, Wang D, Hu W. The emerging role of toll-like receptor 4 in myocardial inflammation. Cell Death Dis 2016; 7(5):e2234. doi: 10.1038/cddis.2016.140 [Crossref] [ Google Scholar]
- Wu S, Zou MH. AMPK, mitochondrial function, and cardiovascular disease. Int J Mol Sci 2020; 21(14):4987. doi: 10.3390/ijms21144987 [Crossref] [ Google Scholar]
- Kahn BB, Alquier T, Carling D, Hardie DG. AMP-activated protein kinase: ancient energy gauge provides clues to modern understanding of metabolism. Cell Metab 2005; 1(1):15-25. doi: 10.1016/j.cmet.2004.12.003 [Crossref] [ Google Scholar]
- Stapleton D, Mitchelhill KI, Gao G, Widmer J, Michell BJ, Teh T. Mammalian AMP-activated protein kinase subfamily. J Biol Chem 1996; 271(2):611-4. doi: 10.1074/jbc.271.2.611 [Crossref] [ Google Scholar]
- Kim M, Tian R. Targeting AMPK for cardiac protection: opportunities and challenges. J Mol Cell Cardiol 2011; 51(4):548-53. doi: 10.1016/j.yjmcc.2010.12.004 [Crossref] [ Google Scholar]
- Kim J, Yang G, Kim Y, Kim J, Ha J. AMPK activators: mechanisms of action and physiological activities. Exp Mol Med 2016; 48(4):e224. doi: 10.1038/emm.2016.16 [Crossref] [ Google Scholar]
- Hardie DG, Ross FA, Hawley SA. AMP-activated protein kinase: a target for drugs both ancient and modern. Chem Biol 2012; 19(10):1222-36. doi: 10.1016/j.chembiol.2012.08.019 [Crossref] [ Google Scholar]
- Zhang L, Frederich M, He H, Balschi JA. Relationship between 5-aminoimidazole-4-carboxamide-ribotide and AMP-activated protein kinase activity in the perfused mouse heart. Am J Physiol Heart Circ Physiol 2006; 290(3):H1235-43. doi: 10.1152/ajpheart.00906.2005 [Crossref] [ Google Scholar]
- Hawley SA, Ross FA, Chevtzoff C, Green KA, Evans A, Fogarty S. Use of cells expressing gamma subunit variants to identify diverse mechanisms of AMPK activation. Cell Metab 2010; 11(6):554-65. doi: 10.1016/j.cmet.2010.04.001 [Crossref] [ Google Scholar]
- Göransson O, McBride A, Hawley SA, Ross FA, Shpiro N, Foretz M. Mechanism of action of A-769662, a valuable tool for activation of AMP-activated protein kinase. J Biol Chem 2007; 282(45):32549-60. doi: 10.1074/jbc.M706536200 [Crossref] [ Google Scholar]
- Hawley SA, Fullerton MD, Ross FA, Schertzer JD, Chevtzoff C, Walker KJ. The ancient drug salicylate directly activates AMP-activated protein kinase. Science 2012; 336(6083):918-22. doi: 10.1126/science.1215327 [Crossref] [ Google Scholar]
- Rencurel F, Foretz M, Kaufmann MR, Stroka D, Looser R, Leclerc I. Stimulation of AMP-activated protein kinase is essential for the induction of drug metabolizing enzymes by phenobarbital in human and mouse liver. Mol Pharmacol 2006; 70(6):1925-34. doi: 10.1124/mol.106.029421 [Crossref] [ Google Scholar]
- Baur JA, Pearson KJ, Price NL, Jamieson HA, Lerin C, Kalra A. Resveratrol improves health and survival of mice on a high-calorie diet. Nature 2006; 444(7117):337-42. doi: 10.1038/nature05354 [Crossref] [ Google Scholar]
- Hwang JT, Park IJ, Shin JI, Lee YK, Lee SK, Baik HW. Genistein, EGCG, and capsaicin inhibit adipocyte differentiation process via activating AMP-activated protein kinase. BiochemBiophys Res Commun 2005; 338(2):694-9. doi: 10.1016/j.bbrc.2005.09.195 [Crossref] [ Google Scholar]
- Lim HW, Lim HY, Wong KP. Uncoupling of oxidative phosphorylation by curcumin: implication of its cellular mechanism of action. BiochemBiophys Res Commun 2009; 389(1):187-92. doi: 10.1016/j.bbrc.2009.08.121 [Crossref] [ Google Scholar]
- Ki SH, Choi JH, Kim CW, Kim SG. Combined metadoxine and garlic oil treatment efficaciously abrogates alcoholic steatosis and CYP2E1 induction in rat liver with restoration of AMPK activity. Chem Biol Interact 2007; 169(2):80-90. doi: 10.1016/j.cbi.2007.05.008 [Crossref] [ Google Scholar]
- Turner N, Li JY, Gosby A, To SW, Cheng Z, Miyoshi H. Berberine and its more biologically available derivative, dihydroberberine, inhibit mitochondrial respiratory complex I: a mechanism for the action of berberine to activate AMP-activated protein kinase and improve insulin action. Diabetes 2008; 57(5):1414-8. doi: 10.2337/db07-1552 [Crossref] [ Google Scholar]
- Lin YC, Hung CM, Tsai JC, Lee JC, Chen YL, Wei CW. Hispidulin potently inhibits human glioblastoma multiforme cells through activation of AMP-activated protein kinase (AMPK). J Agric Food Chem 2010; 58(17):9511-7. doi: 10.1021/jf1019533 [Crossref] [ Google Scholar]
- Pang T, Zhang ZS, Gu M, Qiu BY, Yu LF, Cao PR. Small molecule antagonizes autoinhibition and activates AMP-activated protein kinase in cells. J Biol Chem 2008; 283(23):16051-60. doi: 10.1074/jbc.M710114200 [Crossref] [ Google Scholar]
- Kulkarni SS, Karlsson HK, Szekeres F, Chibalin AV, Krook A, Zierath JR. Suppression of 5’-nucleotidase enzymes promotes AMP-activated protein kinase (AMPK) phosphorylation and metabolism in human and mouse skeletal muscle. J Biol Chem 2011; 286(40):34567-74. doi: 10.1074/jbc.M111.268292 [Crossref] [ Google Scholar]
- Bai A, Yong M, Ma AG, Ma Y, Weiss CR, Guan Q. Novel anti-inflammatory action of 5-aminoimidazole-4-carboxamide ribonucleoside with protective effect in dextran sulfate sodium-induced acute and chronic colitis. J Pharmacol Exp Ther 2010; 333(3):717-25. doi: 10.1124/jpet.109.164954 [Crossref] [ Google Scholar]
- Zhou D, Ai Q, Lin L, Gong X, Ge P, Che Q. 5-aminoimidazole-4-carboxamide-1-β-D-ribofuranoside-attenuates LPS/D-Gal-induced acute hepatitis in mice. Innate Immun 2015; 21(7):698-705. doi: 10.1177/1753425915586231 [Crossref] [ Google Scholar]
- Zhang K, Xu Q, Gao Y, Cao H, Lian Y, Li Z. Polysaccharides from Dicliptera chinensis ameliorate liver disturbance by regulating TLR-4/NF-κB and AMPK/Nrf2 signalling pathways. J Cell Mol Med 2020; 24(11):6397-409. doi: 10.1111/jcmm.15286 [Crossref] [ Google Scholar]
- Prasad R, Giri S, Nath N, Singh I, Singh AK. 5-aminoimidazole-4-carboxamide-1-beta-4-ribofuranoside attenuates experimental autoimmune encephalomyelitis via modulation of endothelial-monocyte interaction. J Neurosci Res 2006; 84(3):614-25. doi: 10.1002/jnr.20953 [Crossref] [ Google Scholar]
- Terkeltaub R, Yang B, Lotz M, Liu-Bryan R. Chondrocyte AMP-activated protein kinase activity suppresses matrix degradation responses to proinflammatory cytokines interleukin-1β and tumor necrosis factor α. Arthritis Rheum 2011; 63(7):1928-37. doi: 10.1002/art.30333 [Crossref] [ Google Scholar]
- Rameshrad M, Soraya H, Maleki-Dizaji N, Vaez H, Garjani A. A-769662, a direct AMPK activator, attenuates lipopolysaccharide-induced acute heart and lung inflammation in rats. Mol Med Rep 2016; 13(3):2843-9. doi: 10.3892/mmr.2016.4821 [Crossref] [ Google Scholar]
- Ghavimi H, Sheidaei S, Vaez H, Zolali E, Asgharian P, Hamishehkar H. Metformin-attenuated sepsis-induced oxidative damages: a novel role for metformin. Iran J Basic Med Sci 2018; 21(5):469-75. doi: 10.22038/ijbms.2018.24610.6126 [Crossref] [ Google Scholar]
- Lei L, Chai Y, Lin H, Chen C, Zhao M, Xiong W. Dihydroquercetin activates AMPK/Nrf2/HO-1 signaling in macrophages and attenuates inflammation in LPS-induced endotoxemic mice. Front Pharmacol 2020; 11:662. doi: 10.3389/fphar.2020.00662 [Crossref] [ Google Scholar]
- Soraya H, Rameshrad M, Mokarizadeh A, Garjani A. Metformin attenuates myocardial remodeling and neutrophil recruitment after myocardial infarction in rat. Bioimpacts 2015; 5(1):3-8. doi: 10.15171/bi.2015.02 [Crossref] [ Google Scholar]
- Zolali E, Shayesteh S, Rahbarghazi R, Vaez H, Heidari HR, Garjani A. Metformin had potential to increase endocan levels in STZ-induced diabetic mice. Pharm Sci 2020; 26(2):133-41. doi: 10.34172/ps.2020.2 [Crossref] [ Google Scholar]
- Lee TH, Park D, Kim YJ, Lee I, Kim S, Oh CT. Lactobacillus salivarius BP121 prevents cisplatin-induced acute kidney injury by inhibition of uremic toxins such as indoxyl sulfate and p-cresol sulfate via alleviating dysbiosis. Int J Mol Med 2020; 45(4):1130-40. doi: 10.3892/ijmm.2020.4495 [Crossref] [ Google Scholar]
- Zhao X, Zmijewski JW, Lorne E, Liu G, Park YJ, Tsuruta Y. Activation of AMPK attenuates neutrophil proinflammatory activity and decreases the severity of acute lung injury. Am J Physiol Lung Cell Mol Physiol 2008; 295(3):L497-504. doi: 10.1152/ajplung.90210.2008 [Crossref] [ Google Scholar]
- Zhu YP, Brown JR, Sag D, Zhang L, Suttles J. Adenosine 5’-monophosphate-activated protein kinase regulates IL-10-mediated anti-inflammatory signaling pathways in macrophages. J Immunol 2015; 194(2):584-94. doi: 10.4049/jimmunol.1401024 [Crossref] [ Google Scholar]
- Salt IP, Palmer TM. Exploiting the anti-inflammatory effects of AMP-activated protein kinase activation. Expert OpinInvestig Drugs 2012; 21(8):1155-67. doi: 10.1517/13543784.2012.696609 [Crossref] [ Google Scholar]
- Eitner A, Müller S, König C, Wilharm A, Raab R, Hofmann GO. Inhibition of inducible nitric oxide synthase prevents IL-1β-induced mitochondrial dysfunction in human chondrocytes. Int J Mol Sci 2021; 22(5):2477. doi: 10.3390/ijms22052477 [Crossref] [ Google Scholar]
- Jeong HW, Hsu KC, Lee JW, Ham M, Huh JY, Shin HJ. Berberine suppresses proinflammatory responses through AMPK activation in macrophages. Am J Physiol Endocrinol Metab 2009; 296(4):E955-64. doi: 10.1152/ajpendo.90599.2008 [Crossref] [ Google Scholar]
- Schuhmacher S, Foretz M, Knorr M, Jansen T, Hortmann M, Wenzel P. α1AMP-activated protein kinase preserves endothelial function during chronic angiotensin II treatment by limiting Nox2 upregulation. ArteriosclerThrombVasc Biol 2011; 31(3):560-6. doi: 10.1161/atvbaha.110.219543 [Crossref] [ Google Scholar]
- Colombo SL, Moncada S. AMPKalpha1 regulates the antioxidant status of vascular endothelial cells. Biochem J 2009; 421(2):163-9. doi: 10.1042/bj20090613 [Crossref] [ Google Scholar]
- Nerstedt A, Johansson A, Andersson CX, Cansby E, Smith U, Mahlapuu M. AMP-activated protein kinase inhibits IL-6-stimulated inflammatory response in human liver cells by suppressing phosphorylation of signal transducer and activator of transcription 3 (STAT3). Diabetologia 2010; 53(11):2406-16. doi: 10.1007/s00125-010-1856-z [Crossref] [ Google Scholar]
- Ewart MA, Kohlhaas CF, Salt IP. Inhibition of tumor necrosis factor alpha-stimulated monocyte adhesion to human aortic endothelial cells by AMP-activated protein kinase. ArteriosclerThrombVasc Biol 2008; 28(12):2255-7. doi: 10.1161/atvbaha.108.175919 [Crossref] [ Google Scholar]
- Buler M, Aatsinki SM, Skoumal R, Komka Z, Tóth M, Kerkelä R. Energy-sensing factors coactivator peroxisome proliferator-activated receptor γ coactivator 1-α (PGC-1α) and AMP-activated protein kinase control expression of inflammatory mediators in liver: induction of interleukin 1 receptor antagonist. J Biol Chem 2012; 287(3):1847-60. doi: 10.1074/jbc.M111.302356 [Crossref] [ Google Scholar]
- Galic S, Fullerton MD, Schertzer JD, Sikkema S, Marcinko K, Walkley CR. Hematopoietic AMPK β1 reduces mouse adipose tissue macrophage inflammation and insulin resistance in obesity. J Clin Invest 2011; 121(12):4903-15. doi: 10.1172/jci58577 [Crossref] [ Google Scholar]
- Nagata D, Hirata Y. The role of AMP-activated protein kinase in the cardiovascular system. Hypertens Res 2010; 33(1):22-8. doi: 10.1038/hr.2009.187 [Crossref] [ Google Scholar]
- Marsin AS, Bertrand L, Rider MH, Deprez J, Beauloye C, Vincent MF. Phosphorylation and activation of heart PFK-2 by AMPK has a role in the stimulation of glycolysis during ischaemia. Curr Biol 2000; 10(20):1247-55. doi: 10.1016/s0960-9822(00)00742-9 [Crossref] [ Google Scholar]
- Kudo N, Barr AJ, Barr RL, Desai S, Lopaschuk GD. High rates of fatty acid oxidation during reperfusion of ischemic hearts are associated with a decrease in malonyl-CoA levels due to an increase in 5’-AMP-activated protein kinase inhibition of acetyl-CoA carboxylase. J Biol Chem 1995; 270(29):17513-20. doi: 10.1074/jbc.270.29.17513 [Crossref] [ Google Scholar]
- Shirwany NA, Zou MH. AMPK in cardiovascular health and disease. Acta Pharmacol Sin 2010; 31(9):1075-84. doi: 10.1038/aps.2010.139 [Crossref] [ Google Scholar]
- Reihill JA, Ewart MA, Hardie DG, Salt IP. AMP-activated protein kinase mediates VEGF-stimulated endothelial NO production. BiochemBiophys Res Commun 2007; 354(4):1084-8. doi: 10.1016/j.bbrc.2007.01.110 [Crossref] [ Google Scholar]
- Versari D, Daghini E, Virdis A, Ghiadoni L, Taddei S. Endothelial dysfunction as a target for prevention of cardiovascular disease. Diabetes Care 2009; 32(Suppl 2):S314-21. doi: 10.2337/dc09-S330 [Crossref] [ Google Scholar]
- Gélinas R, Mailleux F, Dontaine J, Bultot L, Demeulder B, Ginion A. AMPK activation counteracts cardiac hypertrophy by reducing O-GlcNAcylation. Nat Commun 2018; 9(1):374. doi: 10.1038/s41467-017-02795-4 [Crossref] [ Google Scholar]
- Moussa A, Li J. AMPK in myocardial infarction and diabetes: the yin/yang effect. Acta Pharm Sin B 2012; 2(4):368-78. doi: 10.1016/j.apsb.2012.06.001 [Crossref] [ Google Scholar]
- Sidhu JS, Rajawat YS, Rami TG, Gollob MH, Wang Z, Yuan R. Transgenic mouse model of ventricular preexcitation and atrioventricular reentrant tachycardia induced by an AMP-activated protein kinase loss-of-function mutation responsible for Wolff-Parkinson-White syndrome. Circulation 2005; 111(1):21-9. doi: 10.1161/01.cir.0000151291.32974.d5 [Crossref] [ Google Scholar]
- Nagata D, Mogi M, Walsh K. AMP-activated protein kinase (AMPK) signaling in endothelial cells is essential for angiogenesis in response to hypoxic stress. J Biol Chem 2003; 278(33):31000-6. doi: 10.1074/jbc.M300643200 [Crossref] [ Google Scholar]
- Kim AS, Miller EJ, Wright TM, Li J, Qi D, Atsina K. A small molecule AMPK activator protects the heart against ischemia-reperfusion injury. J Mol Cell Cardiol 2011; 51(1):24-32. doi: 10.1016/j.yjmcc.2011.03.003 [Crossref] [ Google Scholar]
- Wyne KL. The effect of hyperglycemia and its therapies on the heart. Heart Fail Clin 2006; 2(1):61-70. doi: 10.1016/j.hfc.2006.01.004 [Crossref] [ Google Scholar]
- Foretz M, Viollet B. Regulation of hepatic metabolism by AMPK. J Hepatol 2011; 54(4):827-9. doi: 10.1016/j.jhep.2010.09.014 [Crossref] [ Google Scholar]
- Kjøbsted R, Hingst JR, Fentz J, Foretz M, Sanz MN, Pehmøller C. AMPK in skeletal muscle function and metabolism. FASEB J 2018; 32(4):1741-77. doi: 10.1096/fj.201700442R [Crossref] [ Google Scholar]
- Hopkins TA, Dyck JR, Lopaschuk GD. AMP-activated protein kinase regulation of fatty acid oxidation in the ischaemic heart. Biochem Soc Trans 2003; 31(Pt 1):207-12. doi: 10.1042/bst0310207 [Crossref] [ Google Scholar]
- Qi D, Young LH. AMPK: energy sensor and survival mechanism in the ischemic heart. Trends Endocrinol Metab 2015; 26(8):422-9. doi: 10.1016/j.tem.2015.05.010 [Crossref] [ Google Scholar]
- Wang M, Li S, Wang F, Zou J, Zhang Y. Aerobic exercise regulates blood lipid and insulin resistance via the toll-like receptor 4-mediated extracellular signal-regulated kinases/AMP-activated protein kinases signaling pathway. Mol Med Rep 2018; 17(6):8339-48. doi: 10.3892/mmr.2018.8863 [Crossref] [ Google Scholar]
- Luna-Vital D, Luzardo-Ocampo I, Cuellar-Nuñez ML, Loarca-Piña G, Gonzalez de Mejia E. Maize extract rich in ferulic acid and anthocyanins prevents high-fat-induced obesity in mice by modulating SIRT1, AMPK and IL-6 associated metabolic and inflammatory pathways. J NutrBiochem 2020; 79:108343. doi: 10.1016/j.jnutbio.2020.108343 [Crossref] [ Google Scholar]
- Kim SY, Jeong S, Jung E, Baik KH, Chang MH, Kim SA. AMP-activated protein kinase-α1 as an activating kinase of TGF-β-activated kinase 1 has a key role in inflammatory signals. Cell Death Dis 2012; 3(7):e357. doi: 10.1038/cddis.2012.95 [Crossref] [ Google Scholar]
- Kim N, Lertnimitphun P, Jiang Y, Tan H, Zhou H, Lu Y. Andrographolide inhibits inflammatory responses in LPS-stimulated macrophages and murine acute colitis through activating AMPK. BiochemPharmacol 2019; 170:113646. doi: 10.1016/j.bcp.2019.113646 [Crossref] [ Google Scholar]
- Liu XM, Peyton KJ, Shebib AR, Wang H, Korthuis RJ, Durante W. Activation of AMPK stimulates heme oxygenase-1 gene expression and human endothelial cell survival. Am J Physiol Heart Circ Physiol 2011; 300(1):H84-93. doi: 10.1152/ajpheart.00749.2010 [Crossref] [ Google Scholar]
- Paine A, Eiz-Vesper B, Blasczyk R, Immenschuh S. Signaling to heme oxygenase-1 and its anti-inflammatory therapeutic potential. BiochemPharmacol 2010; 80(12):1895-903. doi: 10.1016/j.bcp.2010.07.014 [Crossref] [ Google Scholar]
- Rius-Pérez S, Torres-Cuevas I, Millán I, Ortega Á L, Pérez S. PGC-1α, inflammation, and oxidative stress: an integrative view in metabolism. Oxid Med Cell Longev 2020; 2020:1452696. doi: 10.1155/2020/1452696 [Crossref] [ Google Scholar]
- Kim DH, Park MH, Chung KW, Kim MJ, Park D, Lee B. Suppression of FoxO6 by lipopolysaccharide in aged rat liver. Oncotarget 2015; 6(33):34143-57. doi: 10.18632/oncotarget.6219 [Crossref] [ Google Scholar]
- Soraya H, Khorrami A, Garjani A, Maleki-Dizaji N, Garjani A. Acute treatment with metformin improves cardiac function following isoproterenol induced myocardial infarction in rats. Pharmacol Rep 2012; 64(6):1476-84. doi: 10.1016/s1734-1140(12)70945-3 [Crossref] [ Google Scholar]
- Fang Y, Hu J. Toll-like receptor and its roles in myocardial ischemic/reperfusion injury. Med Sci Monit 2011; 17(4):RA100-9. doi: 10.12659/msm.881709 [Crossref] [ Google Scholar]
- Dong B, Qi D, Yang L, Huang Y, Xiao X, Tai N. TLR4 regulates cardiac lipid accumulation and diabetic heart disease in the nonobese diabetic mouse model of type 1 diabetes. Am J Physiol Heart Circ Physiol 2012; 303(6):H732-42. doi: 10.1152/ajpheart.00948.2011 [Crossref] [ Google Scholar]
- Taskin E, Guven C, Tunc Kaya S, Sariman M, Emrence Z, Ekmekci SS. Silencing HMGB1 expression inhibits adriamycin’s heart toxicity via TLR4 dependent manner through MAPK signal transduction. J BUON 2020; 25(1):554-65. [ Google Scholar]
- Tadie JM, Bae HB, Deshane JS, Bell CP, Lazarowski ER, Chaplin DD. Toll-like receptor 4 engagement inhibits adenosine 5’-monophosphate-activated protein kinase activation through a high mobility group box 1 protein-dependent mechanism. Mol Med 2012; 18(1):659-68. doi: 10.2119/molmed.2011.00401 [Crossref] [ Google Scholar]
- Vaez H, Rameshrad M, Najafi M, Barar J, Barzegari A, Garjani A. Cardioprotective effect of metformin in lipopolysaccharide-induced sepsis via suppression of toll-like receptor 4 (TLR4) in heart. Eur J Pharmacol 2016; 772:115-23. doi: 10.1016/j.ejphar.2015.12.030 [Crossref] [ Google Scholar]
- Sun H, Zhu X, Lin W, Zhou Y, Cai W, Qiu L. Interactions of TLR4 and PPARγ, dependent on AMPK signalling pathway contribute to anti-inflammatory effects of vaccariae hypaphorine in endothelial cells. Cell PhysiolBiochem 2017; 42(3):1227-39. doi: 10.1159/000478920 [Crossref] [ Google Scholar]
- Ko HJ, Zhang Z, Jung DY, Jun JY, Ma Z, Jones KE. Nutrient stress activates inflammation and reduces glucose metabolism by suppressing AMP-activated protein kinase in the heart. Diabetes 2009; 58(11):2536-46. doi: 10.2337/db08-1361 [Crossref] [ Google Scholar]
- Federico S, Pozzetti L, Papa A, Carullo G, Gemma S, Butini S. Modulation of the innate immune response by targeting toll-like receptors: a perspective on their agonists and antagonists. J Med Chem 2020; 63(22):13466-513. doi: 10.1021/acs.jmedchem.0c01049 [Crossref] [ Google Scholar]
- Vijay K. Toll-like receptors in immunity and inflammatory diseases: past, present, and future. Int Immunopharmacol 2018; 59:391-412. doi: 10.1016/j.intimp.2018.03.002 [Crossref] [ Google Scholar]